Simulation and Modeling of Turbulent Dispersed-Phase Combustion
Turbulent flows laden with small droplets appear in many environmental and engineering applications. We conduct detailed direct numerical simulations to gain insight into the physical mechanisms and develop models for low-cost LES or RANS simulations.
In particular the interaction of the two phases is not well understood. Droplets disperse driven by fluctuations in the gas velocity and evaporate or condensate driven by fluctuations in the vapor pressure. Persistent clusters of droplets emerge, which in turn influence the lifetime of droplets, mixing of vapor, and overall dynamics of such flows.
Direct Numerical Simulation
On one hand, our approach to the problem are direct numerical simulations (DNS), in which the gas is completely resolved and the droplets are modeled as points. In contrast to flows in nature, our simulations are statistically homogeneous (and stationary). This simplification allows us to conduct well-defined experiments, compile clear statistics, and gain new insights. Three studies of increasing complexity are presented. The studies show the interaction of the two phases with a high degree of accuracy.
Isotropic Turbulence
A first study [external pageGiddey, Meyer, Jenny, Physics of Fluids, 2018call_made] examines droplets that cluster and evaporate in isotropic turbulence. In order to enforce stationarity, turbulence is sustained with linear forcing, droplets that evaporated are replaced, and vapor from the droplets is removed. The simulations reveal clusters, which contain significantly more droplets and vapor than the surrounding gas (see video). Spatial spectra and joint density functions of the vapor content, evaporation rate, and droplet number density show how the size and composition of clusters change with the mass loading, Stokes number, and Reynolds number.
Shear Turbulence
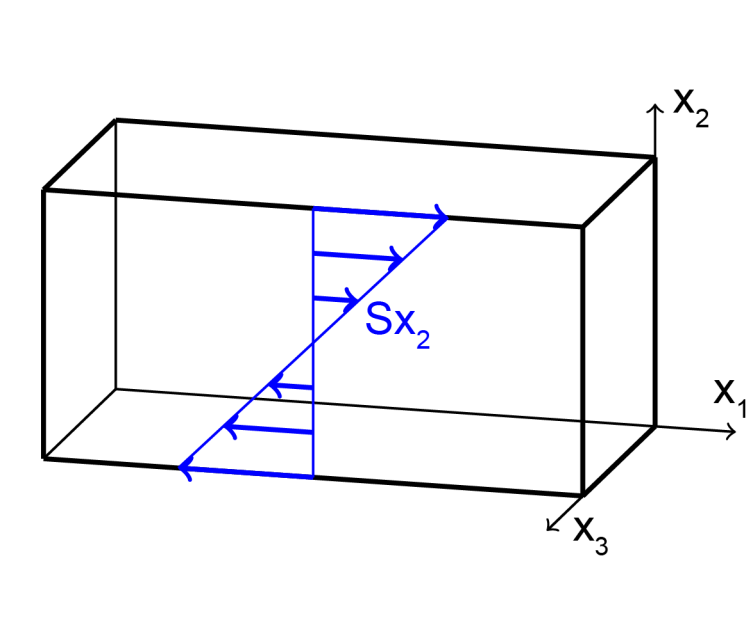
A second study [external pageWeiss, Giddey, Meyer, Jenny, Physics of Fluids, 2020call_made] examines droplets that cluster and evaporate in shear turbulence (3d setup shown in the figure). The simulations are stationary but not isotropic. Like in nature, turbulence is sustained with a mean velocity gradient. As seen in the figure, the simulations reveal anisotropic clusters, which are inclined towards the direction of the mean velocity. Spatial correlation functions of the vapor content and droplet number density show how the inclination of clusters changes with the Stokes number and Reynolds number.
Reactive Turbulence
A third study [external pageWeiss, Bhopalam, Meyer, Jenny, Physics of Fluids, 2021call_made] examines droplets that cluster and evaporate in reactive turbulence. The simulations are isotropic but not stationary. Vapor from the droplets reacts with oxygen in the gas. The simulations reveal diffusion flames, which surround single droplets, and premixed flames, which propagate through clusters. Joint density functions of the reaction rate, flame index, and mixture fraction show how the structure of flames changes with the mass loading and Stokes number.
Model Development
Besides our DNS investigations of dispersed turbulent two-phase combustion, we have developed models for physical effects missing in low-cost spray combustion simulation methods. A first effort was aimed at a turbulence structure model based on the one-dimensional turbulence (ODT) concept [external pageGiddey, Meyer, Jenny, Physics of Fluids, 2018call_made]. This model requires the specification of an eddy-size distribution, which was eliminated by combining ODT with the forcing schemes discussed in connection with [external pageWeiss, Meyer, Jenny, Physics of Fluids, 2018call_made]. In a second step, the resulting ODT model was combined with an analogous one-dimensional scalar description for the mixing of one or two scalars. Comparisons with existing DNS studies were conducted and limitations of the newly-proposed structure-based mixing model discussed.
Our second modeling effort was directly based on our DNS study [external pageWeiss, Oberle, Meyer, Jenny, Physics of Fluids, 2019call_made], where the evaporation of droplets in time- and space-stationary isotropic turbulence was investigated. In low-cost simulation methods, unresolved dynamics of so-called seen gas-phase quantities along particle or droplet paths have to be modeled. While several models have been proposed for seen gas-phase velocities, less work has been done in connection with seen scalars such as species mass fractions or gas temperatures. Based on analytical scaling arguments and our DNS data, models for the integral timescales and Taylor microscales of mass fractions and temperature were developed as a function of the droplet Stokes number and mass loading. Unlike other models, our effort accounts for droplet clustering, which significantly affects these timescales. Furthermore, the mass loading dependency postulated in one of our earlier models for the seen gas-phase velocity has been verified successfully.